How Oxygen Works in Wound Healing
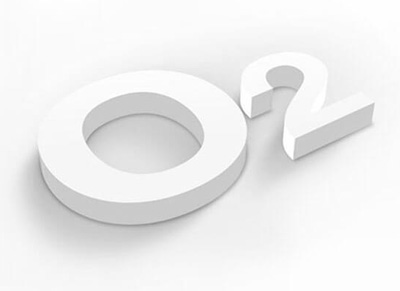
1. Increases Cell Metabolism and Energy
Oxygen is required for intracellular processes like biosynthesis, movement, and transport need energy to be functional, as well as for cell survival (2)
- Oxygen dependent enzymes include:
- Adenosine triphosphate (ATP) for chemical energy, which fuels most active cellular processes such as during wound healing. (3) Increased energy demand of the healing tissue leads to a hypermetabolic state wherein additional energy is generated from oxidative metabolism increasing the oxygen demand of the healing tissue. (4,5,6,7) ATP thus generated powers tissue repair
- NADPH (nicotinamide adenine dinucleotide phosphate) oxygenase for respiratory burst (reactive oxygen species release), the activity of which is critically required to produce the redox signals required for wound healing (8,9,10)
- Aerobic glycolysis, β-oxidation of fatty acids, and the citric acid cycle are tightly attached to the energy acquisition by oxidative phosphorylation and are therefore oxygen dependent (11)
- If oxygen levels are too low (<20 mmHg pO2), cells convert to anaerobic metabolism and go into survival mode in which wound healing activities such as mitosis (cell division, and therefore reepithelialization) and collagen production are impaired (12,13,14)
- Prolonged exposure to extremely low oxygen levels, if not alleviated by oxygen, can result in cell death and tissue necrosis due to the inability of the cells to repair the spontaneous decay of cell components (DNA, RNA, proteins) and inability to maintain calcium pumps which require ATP to function (15,16)
2. Faster Wound Closure (up to 4.6x)
Increasing oxygen levels results in faster cell proliferation, reepithelialization and collagen formation
- The continuous, topical addition of pure oxygen (CDO therapy) over diabetic wounds has been shown to increase the rate of wound closure, by as much as 460% relative to moist wound therapy (17,18,19,20,61)
- Adding oxygen improves closure in wounds that are larger, more chronic and weight-bearing, (17,18) as well as those that are deeper (21)
- CDO therapy has been shown to achieve full wound healing in a variety of wounds that were resistant to other advanced therapy treatments (17,18,19,20,21,22,23,30,31)
- CDO has been shown to increase growth factors (TGF-β, VEGF, PDGF & IGF-1) and cytokines (TNF-α, IL-6 & CXCL8) involved in cell proliferation, reepithelialization and collagen formation in excess of 800% in one week (24)
- Fibroblast proliferation and protein production have been reported to be optimal at 160 mmHg, i.e. at pO2 levels 2-fold to 3-fold higher than those found in healthy tissues (25), indicating that supplemental oxygen increases the rate of wound repair
- Endothelial progenitor cells (EPCs) are essential in wound healing, but their circulating and wound level numbers are decreased in diabetes. Elevated oxygen levels (hyperoxia) reverse the diabetic defect in EPC mobilization (26)
- EPC mobilization into circulation is triggered by hyperoxia through induction of nitric oxide (NO) with resulting enhancement in ischemic limb perfusion and wound healing (27,28,29)
3. Rapid Pain Relief (as fast as several hours)
Increasing oxygen levels relieves hypoxic pain resulting in significant pain reduction –patients have been reported to experience complete pain relief within several hours
- The addition of a continuous flow of pure oxygen over a variety of wound types has been shown to significantly decrease pain in short time frames – over half of the 20 patients experienced at least a 75% reduction within 4 days (30)
- With a patient serving as her own control, CDO had reduced pain from 8 to 2 and she had ceased pain medication. She removed CDO for a few days and the pain increased to 10. Upon reapplying CDO, pain quickly reduced to 2 again. (31)
- In a study of 10 patients with venous ulcers, CDO therapy was reported to significantly (P < .009) reduce pain in a six-week period. The corresponding mean reduction in wound size was 58.9%. (32)
- Several case series reviews report significant pain reduction in very painful wounds. (33,34)
4. Greater Wound Profusion & Angiogenesis
The creation of new blood vessels, angiogenesis, is essential to the growth and survival of repair tissue. Oxygen levels directly affect the rate and quality of new blood vessel growth
- Sufficient oxygen levels are required for correct collagen synthesis (posttranslational hydroxylation) (35), without which the new capillary tubes assemble poorly and remain fragile (36,37,38)
- Supplemental oxygen accelerates blood vessel growth (39)
- Moderate hyperoxia increases the appearance of new blood vessels in wounds (40)
- The rate of angiogenesis is directly proportional to oxygen levels in injured tissues and rates of collagen deposition increase proportionally with oxygen levels to more than 250 mmHg (37)
- Conversely, hypoxic wounds deposit collagen poorly and become infected easily (41,42)
5. Naturally Antibacterial
Oxygen is essential for respiratory burst, the production of reactive oxygen species (ROS), used by phagocytes such as neutrophils and macrophages in bactericidal activity and the removal of necrotic cellular debris
- NADPH oxidase, also known as leukocyte oxidase, supports macrophage survival (delay of apoptosis) (43) and enables dead cell cleansing by phagocytosis (44)
- NADPH oxidase in wound phagocytes, such as neutrophils and macrophages, produces superoxides (O2 and H2O2) for bactericidal activities (45) – in fact, ~98% of oxygen consumed by these cells is used to produce ROS during phagocytosis (46)
- Leukocyte activity (production of ROS and hence oxidative killing) is directly proportional to local oxygen concentration (47,48)
- Optimal ROS production is seen at oxygen levels of greater than 300 mmHg (19), levels which can only be achieved with supplemental oxygen (49)
- At the wound site, ROS are generated by almost all wound-related cells (53)
- The efficacy of supplemental oxygen has been shown to be similar to antibiotic administration and has additive effects when used together (50,51)
6. Better Strength & Appearance
Oxygen is essential to make and properly organize collagen, which is the primary component of skin, accounting for 70-80% (dry weight – without water) and acts as the structural scaffold of skin. Organized collagen is bundled into fibers (like strands in rope), which are interwoven and can be stretched in multiple directions without tearing (the collagen fibers are woven similar to fabric)
- Oxygen is required for the hydroxylation of proline and lysine in procollagen (52)
- Several posttranslational steps in collagen synthesis are oxygen dependent. The enzymes prolyl hydroxylase, lysyl hydroxylase and lysyl oxidase all require oxygen (53,54,55)
- Formation of cross-linked triple-helices via the oxygen-dependent enzyme prolyl hydroxylase and excreted as collagen fibers
- Collagen fibers are arranged into linear fibrils via cross-linking by lysyl hydroxylase
- Linear fibrils are cross-linked by lysyl oxidase – a necessary step to achieve the necessary tensile strength for healed wounds
- Higher oxygen concentrations increase the amount of collagen deposition (42) and tensile strength (56,57,58)
- The rate limiting step is the rate of prolyl hydroxylation (54,55)
- The oxygen level required for optimal prolyl hydryoxlase activity is at oxygen levels approaching 250 mmHg, exceeding those present in normal wounds (59,60)
- CDO has been shown to increase growth factors (TGF-β & VEGF) and cytokines (TNF-α & IL-6) involved in collagen synthesis & remodeling in excess of 800% in one week (24)
- It has been shown that increasing oxygen above normal physiologic levels enhances collagen synthesis and tensile strength in both animal and human subjects (56,57,58) and can increase the level of collagen organization (61)
- Correction of vasoconstriction and hypoxia can result in a 10-fold increase in collagen deposition in wound repair (42,57,62,63)
7. Promotes Growth Factor Signaling Transduction
Reactive oxygen species (ROS) are essential for the signaling processes of growth factors and processes such as leukocyte recruitment, cell motility, angiogenesis and extracellular matrix formation
- Signal transduction of growth factors happens through ROS (64)
- ROS such as hydrogen peroxide (H2O2) increase vascular endothelial growth factor (VEGF) production in macrophages and keratinocytes (65,66)
- VEGF is a major long-term angiogenic stimulus at the wound site
- oxygen treatment induces VEGF mRNA levels in endothelial cells and macrophages (67,68,69)
- oxygen treatment increases VEGF121/165 protein expression in wounds (68) and facilitates the release of VEGF165 from cell-associated stores (71)
- Platelet-derived growth factor (PDGF) requires ROS in its role to regulate cell growth and division (72), and PDGF plays a significant role in blood vessel formation (angiogenesis) (53)
- ROS has effects on other processes such as cytokine action, cell motility and extracellular matrix formation (8)
- CDO has been shown to increase growth factors (TGF-β, VEGF, PDGF & IGF-1) and cytokines (TNF-α, IL-6 & CXCL8) involved in signaling transduction in excess of 800% in one week (24)
- Conversely, tissue hypoxia will limit redox signaling and disable the function of several growth factors (e.g., PDGF, VEGF, keratinocyte growth factor, insulin-like growth factor, transforming growth factor-a) and numerous molecular mechanisms (e.g., leukocyte recruitment, cell motility, integrin function), which rely on redox signaling (10,73,74)
NOTES
- Text from some citations is directly extracted from the referenced literature without quotations
- Recommended summary articles on oxygen in wound care include articles by Sen (2), Tandara and Mustoe (11), Gordillo and Sen (53) and Schreml et al (75)
REFERENCES
- EO2 White Paper: How CDO Works. p/n 690023 (current revision)
- Sen CK. Wound Healing Essentials: Let There Be Oxygen. Wound Rep Reg 2009; 17: 1–18.
- Ichioka S, Ando T, Shibata M, Sekiya N, Nakatsuka T. Oxygen consumption of keloids and hypertrophic scars. Ann Plast Surg 2008; 60: 194–197.
- Gupta A, Raghubir R. Energy metabolism in the granulation tissue of diabetic rats during cutaneous wound healing. Mol Cell Biochem 2005; 270: 71–77.
- Hohn DC, Ponce B, Burton RW, Hunt TK. Antimicrobial systems of the surgical wound. I. A comparison of oxidative metabolism and microbicidal capacity of phagocytes from wounds and from peripheral blood. Am J Surg 1977; 133: 597–600.
- Matsuda T, Clark N, Hariyani GD, Bryant RS, Hanumadass ML, Kagan RJ. The effect of burn wound size on resting energy expenditure. J Trauma 1987; 27: 115–118.
- Im MJ, Hoopes JE. Energy metabolism in healing skin wounds. J Surg Res 1970; 10: 459–464.
- Sen CK. The general case for redox control of wound repair. Wound Repair Regen 2003; 11: 431–438.
- Roy S, Khanna S, Nallu K, Hunt TK, Sen CK. Dermal wound healing is subject to redox control. Mol Ther 2006; 13: 211–220.
- Sen CK, Roy S. Redox signals in wound healing. Biochim Biophys Acta 2008; 1780: 1348–1361.
- Tandara AA, Mustoe TA. Oxygen in Wound Healing – More Than a Nutrient. World J Surg 2004; 28: 294–300.
- LaVan FB, Hunt TK. Oxygen and wound healing. Clin Plast Surg 1990; 17: 463-472.
- Hess CL, Howard MA, Attinger CE. A review of mechanical adjuncts in wound healing: hydrotherapy, ultrasound, negative pressure therapy, hyperbaric oxygen, and electrostimulation. Ann Plast Surg 2003; 51: 210-218.
- Hunt TK. Basic principles of wound healing. J Trauma 1990; 30: S122-S128.
- Milton SL, Prentice HM. Beyond anoxia: The physiology of metabolic downregulation and recovery in the anoxia-tolerant turtle. Comparative Biochemistry and Physiology, Part A 2007; 147: 277–290.
- Stys PK, Ransom BR, Waxman SG, Davis PK. Role of extracellular calcium in anoxic injury of mammalian central white matter. Proc Natl Acad Sci 1990; 87: 4212-4216.
- Niederauer MQ, Michalek JE, Armstrong DG. A Prospective, Randomized, Double-Blind Multicenter Study Comparing Continuous Diffusion of Oxygen Therapy to Sham Therapy in the Treatment of Diabetic Foot Ulcers. J Diabetes Science Tech. 2017, Special Issue, 1-9. DOI: 1h0t.t1p1s:7//7d/o1i.9o3rg2/1209.6118717/1693525279468
- Niederauer MQ, Michalek JE, Liu Q, Papas, Lavery LA, Armstrong DG. Continuous diffusion of oxygen improves diabetic foot ulcer healing when compared with a placebo control: a randomised, double-blind, multicentre study. J Wound Care 27(9):s30-s45 2018.
- Lavery LA, Niederauer MQ, Papas KK, Armstrong DG, Does Debridement Improve Clinical Outcomes in People with DFU Ulcers Treated with CDO? Wounds 31(10):246-251 2019. Epub 2019 July 31
- Chan BCF, Campbell, KE, An economic evaluation examining the cost effectiveness of continuous diffusion of oxygen therapy for individuals with diabeticfootulcers.IntWoundJ.2020;1 18.https://doi.org/10.1111/iwj.13468
- Yu J, Lu S, McLaren A, Perry JA, Cross KM. Topical oxygen therapy results in complete wound healing in diabetic foot ulcers. Wound Rep Regen. 2016;24(6):1066-1072.
- Urrea-Botero G. Can continuous diffusion of oxygen heal chronic toe ulcers? Podiatry Today. 2015;28(10).
- Couture M. Does continuous diffusion of oxygen have potential in chronic diabetic foot ulcers? Podiatry Today. 2015;28(12).
- Lavery LA, Killeen AL, Farrar D, Akgul Y, Crisologo PA, Malone M, Davis KE. The effect of continuous diffusion of oxygen treatment on cytokines, perfusion, bacterial load, and healing in patients with diabetic foot ulcers. Int Wound J. 2020;1–10. https://doi.org/10.1111/iwj.13490
- Pandit AS, Faldman DS. Effect of oxygen treatment and dressing oxygen permeability on wound healing. Wound Repair Regen 1994; 2: 130-137.
- Gallagher KA, Liu ZJ, Xiao M, Chen H, Goldstein LJ, Buerk DG, Nedeau A, Thom SR, Velazquez OC. Diabetic impairments in NO-mediated endothelial progenitor cell mobilization and homing are reversed by hyperoxia and SDF-1 alpha. J Clin Invest 2007; 117: 1249–1259.
- Goldstein LJ, Gallagher KA, Bauer SM, Bauer RJ, Baireddy V, Liu ZJ, Buerk DG, Thom SR, Velazquez OC. Endothelial progenitor cell release into circulation is triggered by hyperoxia-induced increases in bone marrow nitric oxide. Stem Cells 2006; 24: 2309–2318.
- Gallagher KA, Goldstein LJ, Thom SR, Velazquez OC. Hyperbaric oxygen and bone marrow-derived endothelial progenitor cells in diabetic wound healing. Vascular 2006; 14: 328–337.
- Thom SR, Bhopale VM, Velazquez OC, Goldstein LJ, Thom LH, Buerk DG. Stem cell mobilization by hyperbaric oxygen. Am J Physiol Heart Circ Physiol 2006; 290: H1378–H1386.
- Bowen J, Ingersoll MS, Carlson R. Effect of CDO on Pain in Treatment of Chronic Wounds. Wound Central 2(4);186-195 2018.
- Brannick B, Engelthaler M, Jadzak J, Wu S. A closer look at continuous diffusion of oxygen therapy for a chronic, painful venous leg ulcer. Podiatry Today. 2014;27(11).
- Mani R. Topical oxygen therapy for chronic wounds: a report on the potential of Inotec, a new device for delivering oxygen to chronic wounds. J Wound Technol. 2010;9:1-4.
- Lowell DL, Nicklas B, Weeily W, Johnson F, Lyons MC. Transdermal continuous oxygen therapy as an adjunct for treatment of recalcitrant and painful wounds. Foot Ankle Online J. 2009;2(9):4.
- Hirsh F, Berlin SJ, Holtz A. Transdermal oxygen delivery to wounds: a report of 6 cases. Adv Skin Wound Care. 2008;22:20-24.
- Mussini E, Hutton JJ, Jr. Udenfriend S. Collagen proline hydroxylase in wound healing, granuloma formation, scurvy, and growth. Science 1967; 157: 927–929.
- Berthod F, Germain L, Tremblay N, Auger FA. Extracellular matrix deposition by fibroblasts is necessary to promote capillary-like tube formation in vitro. J Cell Physiol 2006; 207: 491–498.
- Hopf HW, Gibson JJ, Angeles AP, Constant JS, Feng JJ, Rollins MD, Zamirul Hussain M, Hunt TK. Hyperoxia and angiogenesis. Wound Repair Regen 2005; 13: 558–564.
- Hunt TK, Aslam RS, Beckert S, Wagner S, Ghani QP, Hussain MZ, Roy S, Sen CK. Aerobically derived lactate stimulates revascularization and tissue repair via redox mechanisms. Antioxid Redox Signal 2007; 9: 1115–1124.
- Knighton D, Silver I, Hunt T. Regulation of wound healing and angiogenesis—effect of oxygen gradients and inspired oxygen concentrations. Surgery 1981; 90: 262–270.
- Sheikh AY, Rollins MD, Hopf HW, Hunt TK. Hyperoxia improves microvascular perfusion in a murine wound model. Wound Repair Regen 2005; 13: 303–308.
- Hunt TK, Zederfeldt B, Goldstick TK. Oxygen and healing. Am J Surg 1969; 118: 521–525.
- Jonsson K, Jensen J, Goodson W, Scheuenstuhl H, West J, Hopf H, Hunt T. Tissue oxygenation, anemia, and perfusion in relation to wound healing in surgical patients. Ann Surg 1991; 214: 605–613.
- Wang Y, Zeigler MM, Lam GK, Hunter MG, Eubank TD, Khramtsov VV, Tridandapani S, Sen CK, Marsh CB. The role of the NADPH oxidase complex, p38 MAPK, and Akt in regulating human monocyte/macrophage survival. Am J Respir Cell Mol Biol 2007; 36: 68–77.
- Brown JR, Goldblatt D, Buddle J, Morton L, Thrasher AJ. Diminished production of anti-inflammatory mediators during neutrophil apoptosis and macrophage phagocytosis in chronic granulomatous disease (CGD). J Leukoc Biol 2003; 73: 591–599.
- Babior BM. Oxygen-dependent microbial killing by phagocytes (first of two parts). N Engl J Med 1978; 298: 659–668.
- Allen DB, Maguire JJ, Mahdavian M, Wicke C, Marcocci L, Scheuenstuhl H, Chang M, Le AX, Hopf HW, Hunt TK. Wound hypoxia and acidosis limit neutrophil bacterial killing mechanisms. Arch Surg 1997; 132: 991–996.
- Rabkin JM, Hunt TK. Infection and oxygen. In Davis JC, Hunt TK, editors, Problem Wounds: The Role of Oxygen, New York, Elsevier, 1988: 1–16.
- Cianci P. Advances in the treatment of the diabetic foot: Is there a role for adjunctive hyperbaric oxygen therapy? Wound Repair Regen 2004;12(1):2-10.
- Wattel F, Mathieu D. Oxygen and wound healing. Bull Acad Natl Med 2005; 189: 853–864.
- Knighton D, Halliday B, Hunt T. Oxygen as an antibiotic: the effect of inspired oxygen on infection. Arch Surg 1984; 119: 199–204.
- Knighton D, Halliday B, Hunt T. Oxygen as an antibiotic. Arch Surg 1986; 121: 191–195.
- Hunt TK, Zederfeldt B, Goldstick TK. Oxygen and healing. Am J Surg 1969; 118: 521–525.
- Gordillo GM, Sen CK. Revisiting the Essential Role of Oxygen in Wound Healing. Amer J Surg 2003; 186: 259–263.
- Prockop D, Kivirikko K, Tuderman L, Guzman N. The biosynthesis of collagen and its disorders (part 1). N Engl J Med 1979; 301: 13–23.
- Prockop D, Kivirikko K, Tuderman L, Guzman N. The biosynthesis of collagen and its disorder (part 2). N Engl J Med 1979; 301: 77–85.
- Niinikoski J. Effect of oxygen supply on wound healing and formation of experimental granulation tissue. Acta Physiol Scand 1970; 78: 1–72.
- Hunt T, Pai M. The effect of varying ambient oxygen tensions on wound metabolism and collagen synthesis. Surg Gynecol Obstet 1972; 135: 561–567.
- Stephens F, Hunt T. Effect of changes in inspired oxygen and carbon dioxide tensions on wound tensile strength. Ann Surg 1971; 173: 515.
- Hutton J, Tappel A, Undenfried S. Cofactor and substrate requirements of collagen proline hydroxylase. Arch Biochem Biophys 1967; 118: 231–240.
- Myllyla R, Tuderman L, Kivirikko K. Mechanism of the prolyl hydroxlase reaction. 2. Kinetic analysis of the reaction sequence. Eur J Biochem 1977; 80: 349–357.
- Asmis R, Qiao M, Zhao Q. Low-Flow Oxygenation of Full-Excisional Skin Wounds on Diabetic Mice Improves Wound Healing by Accelerating Wound Closure and Reepithelialization. Int Wound J 2010 ; 7: 349-357.
- Hopf H, Hunt T, West J, et al. Wound tissue oxygen tension predicts the risk of wound infection in surgical patients. Arch Surg 1997; 132: 997–1004.
- Hartmann M, Jonsson K, Zederfeldt B. Effect of tissue perfusion and oxygenation on accumulation of collagen in healing wounds. Randomized study in patients after major abdominal operations. Eur J Surg 1992; 158: 521–526.
- Sundaresan M, Yu ZX, Ferrans VJ, et al. Regulation of reactive oxygen species generation in fibroblasts by Rac1. Biochem J 1996; 318: 379–382.
- Sen CK, Khanna S, Babior BM, et al. Oxidant-induced vascular endothelial growth factor expression in human keratinocytes and cutaneous wound healing. J Biol Chem 2002; 277: 33284–33290.
- Cho M, Hunt TK, Hussain MZ. Hydrogen peroxide stimulates macrophage vascular endothelial growth factor release. Am J Physiol Heart Circ Physiol 2001; 280: H2357–H2363.
- Maniscalco WM, Watkins RH, Finkelstein JN, Campbell MH. Vascular endothelial growth factor mRNA increases in alveolar epithelial cells during recovery from oxygen injury. Am J Respir Cell Mol Biol 1995; 13: 377–386.
- Deaton P, McKellar C, Culbreth R, et al. Hyperoxia stimulates interleukin-8 release from alveolar macrophages and U937 cells: attenuation by dexamethasone. Am J Physiol 1994; 267: L187–192.
- Darrington R, Godden D, Park M, et al. The effect of hyperoxia on expression of cytokine mRNA in endothelial cells. Biochem Soc Trans 1997; 25: 2925.
- Sheikh A, Gibson J, Rollins M, et al. Effect of hyperoxia on vascular endothelial growth factor levels in a wound model. Arch Surg 2000;153:1293–1297.
- Shenberger JS, Zhang L, Powell RJ, Barchowsky A. Hyperoxia enhances VEGF release from A549 cells via post-transcriptional processes. Free Radic Biol Med 2007; 43: 844–852.
- Sundaresan M, Yu ZX, Ferrans VJ, et al. Requirement for generation of H2O2 for platelet-derived growth factor signal transduction. Science 1995; 270: 296–9.
- Roy S, Khanna S, Sen CK. Redox regulation of the VEGF signaling path and tissue vascularization: hydrogen peroxide, the common link between physical exercise and cutaneous wound healing. Free Radic Biol Med 2008; 44: 180–192.
- Roy S, Khanna S, Rink C, Biswas S, Sen CK. Characterization of the acute temporal changes in excisional murine cutaneous wound inflammation by screening of the wound-edge transcriptome. Physiol Genomics 2008; 34: 162–184.
- Schreml S, Szeimies RM, Prantl L, Karrer S, Landthaler M, Babilas P. Oxygen in acute and chronic wound healing. Brit J Derm 2010; 163: 257-268.